IGE/OGE Hover Performance
- lawebdesignllc
- May 6, 2020
- 6 min read
Many pilots attending the Safety Course at Robinson have asked questions regarding correct use of the hover performance charts in the Performance Section of the Pilot’s Operating Handbook (POH). Let’s use the R44 Raven I OGE chart for our example. As one can easily see, there are three variables involved: pressure altitude, temperature and gross weight. Of these variables there is only one the pilot really has total control of–weight, so that’s what one should solve for. If I were planning to make an off airport or confined area landing at 8000’ PA with a temperature of +10˚C, I would want to know if I had OGE capability. Enter the chart at the 8000’ pressure altitude point on the left side of the chart, move right to intersect the +10˚C temperature line then down to the gross weight. So, at 8000’PA, +10˚C the helicopter can hover OGE at weights up to 1920 lbs (870 kg). If my actual weight is more than 1920 lbs, I know exactly how much weight to remove by reducing fuel, baggage or even passengers. Entering the chart at the gross weight then working backwards may become a trial-and-error process incrementally reducing the weight until OGE capability is assured.
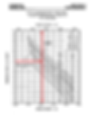
Some pilots want to enter the chart at the gross weight they’ve calculated for a particular flight to find what the OGE hover ceiling is for this flight. The only problem here is that the hover ceiling will vary with temperature. Options include using the dashed standard day temperature line on the chart which starts at sea level standard temperature (+15ºC) and decreases 2˚ per 1000 ft of altitude. Or one could apply this lapse rate beginning at the takeoff temperature. Some pilots incorrectly just use the departure airport temperature. In any case the answer is not really worth very much. How valuable is it to know that your hover ceiling for a particular flight is 6000’ if the intended landing is at 3000 ft? What you really need to know is what is the performance at 3000 f ft. However, what could be worthwhile is entering the chart at the gross weight if you are in flight and know the temperature (from the OAT gauge) and know the weight (A good reason to know the “zero fuel weight” so the weight of the fuel onboard at a particular time can be added to it to determine the changing gross weight as the fuel is burned.) and you need to determine the pressure altitude for OGE capability in order to select a landing area.
Pilots should understand that wind and use of carburetor heat are not accounted for in the hover charts. Winds can obviously hurt or help the condition depending on the direction and velocity. Use of carburetor heat increases the temperature of the air going into the engine thus reducing the engine’s ability to produce horsepower. The R22 and R44 Raven I POHs have a note in the Performance Section telling the pilot that full carburetor heat reduces hover ceiling by as much as 2000 ft (R22) or 2400 ft (R44). These values were not determined by actual flight tests, rather derived using a Lycoming horsepower versus temperature chart for each engine and represent the worst case. Less severe conditions or partial heat may reduce this number but a safe practice is to apply these altitude reductions when using carburetor heat.
Hovering in-ground- effect is normally considered being an altitude within one rotor diameter from the ground. The R22 rotor diameter is approximately 25 feet (actually 25’2”), so when the R22 rotor disk is within 25 feet of the ground the helicopter is in-ground-effect. Understand it’s the rotor disk not the skid height. The R22 rotor is approximately 9 feet (107 inches) high, so the maximum skid height for an IGE hover is 16 feet (25-9=16). The R44 rotor diameter is 33 feet and the disk sits a little more than 11 feet above the ground (129 inches) making the maximum IGE skid height 22 feet. However, the IGE hover chart assumes only a two-foot skid height (see note at the top of the chart). Consequently, any IGE hover altitude above two feet will result in reduced hover capability, something pilots need to keep in mind when operating near the hover limits.
The out-of-ground effect altitude is tested at hover heights equivalent to 1 ½ times the rotor diameter. The method Robinson uses to insure the helicopter stays at or above this required altitude during the test is by using an appropriate length long line with a weight at the end. During the hover test the weight is not permitted to touch the ground. I should note that for older performance data (R22 and R44 I), FAA guidance was to use a height of 1 ½ rotor diameters but did not specify details, so the rotor disk was placed 1 ½ rotor diameters high. More recent guidance specifies that the bottom of the “vehicle” (skid gear) must be 1 ½ rotor diameters high making the R44 II and R66 data slightly less optimistic.

I am also frequently asked the reason for the “dogleg” or “kink” in the temperature lines, especially on many of the OGE charts. Remember that the engines are derated to increase altitude capability. So, using the R44 OGE chart above, if we start at 2300 lbs and move up the +40∞line initially our limiting factor is manifold pressure or takeoff horsepower and the performance reduction with altitude is not too great. Here the engine can produce more horsepower than our 5-minute limit but we are limited to just the 5-minute rating, so horsepower remains constant as altitude increases. When we reach the dogleg at approximately 1800 ft, we are at the point where now the engine is only capability of producing the 5-minute limit and the full throttle light will be on. Continuing up the +40∞line the limit is now full throttle not manifold pressure and performance decreases more rapidly because now horsepower is decreasing as we continue to climb.
Notice all the hover performance charts stop well short of the aircraft’s maximum operating density altitude of 14,000 ft. 14 CFR §27 (Airworthiness Standards–Normal Category Helicopters) allows the manufacture to extrapolate 4000 ft above the density altitude where the test is actually conducted. Robinson uses Big Bear, California, elevation 6752 ft, for most of its high-altitude testing. The day the test was conducted in the Beta II the density altitude at Big Bear was 8600 ft, add 4000 ft and the chart ends at 12,600 ft density altitude. The day the Raven I test was conducted it was a little cooler at Big Bear so the density altitude was 7600 ft, resulting in the chart ending at 11,600 ft density altitude. If Robinson wanted the hover performance charts to extend all the way up to the maximum operating density altitude, the test would have to be conducted at 10,000 ft density (probably Lake County Airport outside of Leadville, Colorado with an airport elevation of 9934 ft, which is the highest in the US) then Robinson engineers could extrapolate up to 14,000 density.
There is also a difference between the R22, Raven I and Raven II hover performance charts that reflect a change to 14 CFR §27 after the R22 was certified but before the R44 was approved, followed by a change in the interpretation of the rule. When the R22 was certified in 1979, there was no requirement to account for the effects of humidity in the hover performance data. Whereas, when the R44 was certified in late 1992 (the Astro model) the rule had changed so that the effect of an 80% relative humidity was calculated into the data. This was stated in early versions of the R44 POH. Higher humidity means more water in the air resulting in less oxygen which reduces the engine’s ability to produce horsepower. The R44 Astro/Raven I hover charts use this 80% humidity factor throughout the temperature range which is why there is a slight curve in the density altitude line, whereas in the R22 charts, which do not consider humidity, the line is straight. Note this curvature on the Astro/Raven I charts is slightly more pronounced at the higher temperatures. This is because the air’s ability to retain water increases with temperature. So, the amount of water in the air at 80% relative humidity and 10ºC is less than at 80% relative humidity and 40ºC, resulting in the slight decrease in performance as the curve indicates. However, a different interpretation was used for the Raven II. The rule says 80% relative humidity in a standard atmosphere so Robinson used sea level, pressure 29.92 in hg (1013.2 mb) and +15ºC to calculate the humidity or the amount of water in the air and used that amount throughout the Raven II charts. This essentially eliminated the curvature in both the Raven II IGE and OGE charts.
Tim Tucker
November 2018