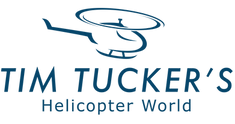
My Take on Autorotations
Tim Tucker

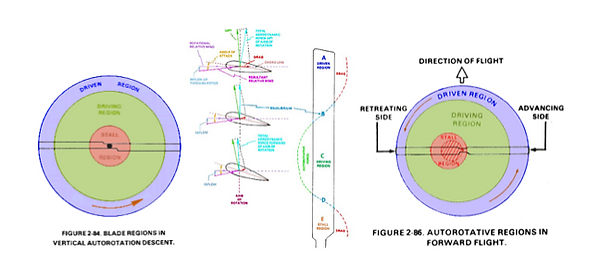
Introduction
In the entire helicopter lexicon there is certainly no other term that has provoked more discussion, arguments, controversy and perhaps even a bar fight or two than the word “autorotation”. From aircraft flight testing to pilot flight training to that time during a pleasant flight when all of a sudden it gets real quiet inside the cockpit, the concept of an autorotation occupies more of a helicopter pilot’s thinking than just about anything else.
I clearly remember when I first heard the word “autorotation”. I had just stepped off a bus with a bunch of other US Army basic training graduates after an all-day trip from Ft. Polk, Louisiana to Ft Wolters, Texas to begin Army flight training. As we double timed into a formation for roll call, we could see on the horizon above the tree-line numerous training helicopters, the one we would soon be flying (the Army TH-55 Osage). It riveted our attention. Each helicopter got to a certain spot above a tree then seemed to just fall straight down out of the sky at an alarming rate. I couldn’t see the termination because of the trees but I thought they had crashed although there was no smoke or noise and no one seemed overly concerned. The Warrant Officer candidate standing next to me noticed my concern and confidently said “they are practicing autorotations”. I swallowed and thought to myself maybe the infantry would have been a better choice for my Army experience.
Five weeks later, after what the Army called “pre-flight” which consisted of a combination of academics and general harassment (the Army referred to it as “officer training”), my instructor pilot demonstrated my first autorotation. At that time in the Army, all autorotations were to the ground–no power recoveries even in the low inertia TH-55 (the civilian equivalent is the HU269A). Things seemed to happen so fast it was a blur–the ground rushing up at 2000 ft/min, then just as I thought we were going to crash, the helicopter rotated up so I was staring at the sky, back level with the ground, up collective, a slight bounce as we hit the ground scraping along the stage field runway for 20-30 feet and finally came to a complete stop. A controlled crash is a kind description. However, my instructor turned to me and said “See, nothing to it!”
Energy Management
Any discussion of autorotations has to begin with energy. All a good autorotation really is, is an exercise in knowing where and how to manage and use the available energy. The law of “conservation of energy” states that energy can neither be created nor destroyed and the total amount of energy in an isolated system remains constant. What’s important for a helicopter pilot to understand is when the engine quits; you are now an isolated system. The amount of energy you have available when the engine fails is all you’re going to have. There is nothing you can do to increase it. Bear that in mind when you choose what airspeed and altitude to fly at. However, the energy can change form or be transferred from one area to another. So, for example, one could change mechanical energy to electrical energy or in the helicopter’s case, from potential to kinetic energy.
The helicopter stores two types of energy in flight, kinetic energy and potential energy. Kinetic energy is the energy of speed or motion and is stored in two areas-the speed of the helicopter and the speed of the rotor blades. It is divided into two types: linear kinetic energy from the speed the helicopter and rotational kinetic energy from the spinning rotor. The formulas for calculating these two types of kinetic energy are similar but slightly different (½ MV² versus ½ IΩ²). However, for simplicity’s sake and not cluttering the discussion up with Greek symbols, terms like “moment of inertia” and “angular velocity”, I will consider them the same for our purposes. So, to calculate the kinetic energy in both areas I will use KE = ½ MV², where M is the mass or we can use weight and V is the speed or velocity (speed of the aircraft or speed of the rotor). Since the velocity is being squared changes in velocity will produce large changes in the total amount of kinetic energy, whereas a change in weight will produce a change in the total energy but the change will not be as significant since it is ½ M. For example, this chart shows the amount of forward speed energy, expressed in hp-sec (one hp-sec is the amount of energy to produce one horsepower for one second) an R22 at maximum gross weight (1370 lbs) will produce at various airspeeds.
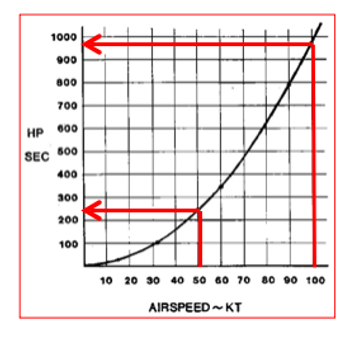
Notice that at 50 kts the forward speed will produce 250 hp-sec of kinetic energy, but if the speed is doubled to 100 kts, the amount of energy produced is much more than doubled, at 970 hp-secs. So, aircraft speed becomes very important in the autorotation. Potential energy is the energy of height and can be calculated using the formula PE = W x H, where W is the aircraft’s weight and H is the height above the ground.
What I will do is take two different flight conditions, again in an R22 at maximum gross weight, analyze the amount of energy available to the pilot then assume the engine fails creating an isolated system. Managing the energy now becomes the pilot’s foremost responsibility and we’ll examine a couple of different options.
The first condition is 90 kts at 500 feet AGL. Here is how the energy breaks down:
Kinetic energy in the rotor RPM - 100 hp-sec
Kinetic energy in the forward speed - 800 hp-sec
Potential energy from altitude - 1100 hp-sec
Total energy - 2000 hp-sec
One word of explanation regarding the kinetic energy in the rotor RPM, if you took ½ the weight of a rotor blade times the speed of the blade squared, then multiplied by two since there are two blades, you would find there is much more than 100 hp-sec of energy in the spinning rotor. The problem comes in accessing or using this energy. Once the rotor RPM decays to a certain point the blade encounters an aerodynamic stall. Once the blade stalls we can no longer access any of the energy the stalled rotor is producing.
So, at 90 kts/500 feet when the engine quits the pilot has 2000 hp-sec of energy to make a successful autorotation landing. Let’s look at the two ends of the spectrum here. First let’s assume the pilot is going to use whatever energy is available to maintain airspeed and altitude. I understand this is probably not going to be a pilot’s fist course of action when the engine really quits but let’s take a look at it. An R22 rotor needs 90 hp to maintain airspeed and altitude but since this pilot is trying to maintain airspeed and altitude, none of the energy stored in these areas can be used. The only place left where energy is being stored is in the rotor RPM where there is only 100 hp-sec. The rotor needs 90 hp, 100 hp-sec is available so all the energy in that spinning rotor will be used up in 1.1 sec (100 hp-sec divided by 90 hp). Understand this is using all the rotor energy by full up collective and most helicopters will be in this general time frame. Variations will occur with different inertia rotor systems (high or low) when the collective is not moved and the RPM is allowed to decay on its own. This underscores the importance of lowering the collective or, at least not, raising the collective when the engine fails. At the other end of the spectrum, if the pilot is allowed to use all 2000 hp-sec of energy and the pilot does everything perfectly (entry, glide, flare and landing), time is not near as critical. In an autorotative glide the R22 rotor only needs 75 hp (no attempt to maintain straight and level). So, 2000 hp-sec divided by 75 hp means the helicopter will be on the ground in 26 seconds. Big difference between 1.1 seconds and 26 seconds.
Now let’s change the condition to 60 kts and only 100 feet AGL. Here is how the energy now breaks down:
Kinetic energy in the rotor RPM - 100 hp-sec
Kinetic energy in the forward speed - 346 hp-sec
Potential energy from altitude - 220 hp-sec
Total energy - 666 hp-sec
Note the large energy reductions in the forward speed and altitude areas. If, again, the pilot attempted to maintain airspeed and altitude after the engine failure it would be the same 1.1 seconds, but if the pilot had all 666 hp-sec available, ground contact is only nine seconds away (666 hp-sec divided by 75 hp). Clearly, from an energy standpoint it’s safer to be higher and faster rather than lower and slower.
Another interesting perspective is to compare the amount of kinetic energy in the rotor RPM to a specific decrease in airspeed. In the R22 a RPM reduction from 104% (top of the green) to 75% uses 100 hp-sec of energy. That’s essentially a hovering autorotation in an R22. That same 100 hp-sec can be recovered with an airspeed reduction from 100 kts to 84 kts – just a six-knot reduction regains the same amount of energy as an entire hovering autorotation. This is the essence of the “cyclic back” technique from Pete Gilles of Western Helicopters. Especially in faster helicopters, the energy from an aft cyclic input (airspeed reduction) can be more important than initially lowering the collective in preventing an excessive rotor decay when the engine fails . However, the slower the airspeed is, the more of an airspeed reduction is needed to recover the same 100 hp-sec. So, in the R22 a reduction from 60 kts to 50 kts or from 32 kts all the way to zero is needed to recover 100 hp-sec. Additionally, for every 46 ft of altitude lost, 100 hp-sec of potential energy is recovered. Again, higher and faster.
Although, I’ve used an R22 for this explanation and the numbers will vary with other helicopters, the theory is the same.
Aerodynamics
I don’t want to spend much time on the aerodynamics of the autorotation as there are numerous good references that explain how the regions of the blade produce the autorotative forces. However, I want to make two points. The rotor RPM is produced by the upward flow of air through the rotor due entirely to the descent rate (converting potential energy to kinetic energy). Other than short-term effects of accelerating or decelerating, forward speed has little to do with this conversion. Consequently, the helicopter autorotates (maintains appropriate rotor RPM) just fine at airspeeds to down to zero, a technique I will explain in the Enhanced Autorotation discussion. Second, a word about the power-off Vne, some helicopters have a power-off Vne because of the inability to maintain rotor RPM within limits in autorotation at high airspeeds. As the airspeed increases the angle of the airflow into the disk becomes more horizontal reducing the size of the autorotative driving region of each blade causing a decay in rotor RPM. Airspeed indicators will have a red and white cross hatched line (like a barber pole) or a blue line at the power-off Vne. Other reasons for a power-off Vne can be controllability issues such as the need for left pedal in the AS-350 or high fatigue loads in autorotation at high speeds, for example the R44 and R66.
New Terminology
With the new Helicopter Airman Certification Standards (ACS) and the corresponding revision to the FAA’s Helicopter Flying Handbook (FAA-8083-H-21B) new terminology will be introduced into the helicopter autorotation vocabulary (as I write this a publishing date has not been determined by the FAA). The “Basic Autorotation” will replace the “Straight-in Autorotation”, “Autorotation With Turn” replaces the “180º Autorotation” and the “Advanced Autorotation” will be added. Terms like “Forced landing”, “Simulated Engine Failure”, “Throttle Chop”, etc. will all be replaced with “Power Failure at Altitude”. I am on the FAA’s ACS Working Group and will try to explain the thinking around some of these changes. The Working Group wanted to move away from the structured specifics of the straight-in/180º mindset. The well-known US Army story of a foreign student’s engine failure during a solo flight is at the heart of this mindset. When asked why he did not turn towards the big field 90º to his left, the response was “Sir, I have only been taught a straight-in and a 180º autorotation”. We wanted to focus more on the use of elements to maneuver the helicopter in autorotation and emphasize accuracy where it’s most appropriate. Therefore, we eliminated the accuracy standard (±200 ft for the private and ±100 ft for the commercial) from basic and auto with turn maneuver in ACS. This accuracy requirement from 500 to 700 ft in the old Practical Test Standard (PTS) was really more of a test to see if the student could determine where to enter the autorotation than any real flying skills and not of much value in the real world. Of course, accuracy and the knowledge/skill to maneuver the helicopter in autorotation is critical to a successful outcome, so we developed the “Advanced Autorotation” where knowledge at the private level and skill at the commercial level are evaluated. We have also added the skill requirement for a “distress” or “mayday call” to the Power Failure at Altitude which I’ll discuss later in this essay. At this point these changes are in the form of recommendations from the Aviation Rulemaking Advisory Committee (ARAC), which consists of both industry and FAA representatives, to the FAA for incorporation into the new Helicopter ACS.
The Basic Autorotation
The Basic Autorotation is just that–basic. Its purpose is to teach or practice the fundamentals of autorotative flight. My discussion will be general and applies to helicopters with a main rotor rotation that is counterclockwise. For recommended airspeeds and specific techniques for a particular make & model the aircraft’s Rotorcraft Flight Manual (RFM), Pilot Operating Handbook (POH) or, with Robinson Helicopters, the Maneuver Guide is the place to go. We can divide the Basic Autorotation into four sections: the entry, the glide, the flare and the landing or power recovery depending to the intent.
The Entry: The purpose of the entry is to get into autorotative flight as easily as possible. It’s not intended to be used to teach recognition of an engine failure that will come later in the Power Failure at Altitude maneuver. Therefore, the student or pilot should enter the autorotation themselves. I frequently find instructors who try to insert a recognition element into the entry which I feel is a mistake. I think the entry is the most important of the four sections because if the entry is screwed up, a whole lot of work is required to clean up the problems created by the poor entry. Whereas, if the entry is smooth and coordinated the pilot can now focus on the next portion of the autorotation rather than immediately fixing problems caused in the entry. From the normal traffic pattern altitude (minimum 500 ft AGL) or higher and the recommended autorotational airspeed plus 5–10 kts, begin the entry by simultaneously lowering the collective full down firmly but smoothly, right pedal to maintain trim and aft cyclic to prevent a pitch down attitude. When to roll the throttle off depends on the make & model of helicopter. In many piston engine helicopters (R22 and R44) and some turbine helicopters, the throttle should be retarded once the collective is full down. However, in other turbine aircraft such as the R66, the N2 will tend to overspeed if the collective is lowered to quickly so the throttle should be reduced prior to lowering the collective. Another note about turbine engines is when the throttle is reduced on the entry, the N1 decays at a set schedule set into the fuel control unit to prevent interrupting the fuel supply to the engine causing a flame out. This additional output torque will cause a much slower RPM decay than if the engine actually failed. One of the more common mistakes I see on a private and even a commercial flight test is failing to apply enough aft cyclic during the entry. In all helicopters when the collective is lowered the nose wants to drop due to the rotor’s forward tilt caused by a decrease in blade angle of attack, the resulting decrease in flapping and gyroscopic precession. This will cause an increase in airspeed and a greater decrease in rotor RPM, the exact opposite of a flare. The energy from a proper aft cyclic input will also help prevent an excessive rotor RPM decay especially at higher airspeeds. As the collective is being lowered, focus on the aircraft attitude (horizon) and apply enough aft cyclic to at least prevent the nose from dropping. Trim is also very important on the entry. There is no other maneuver in the helicopter whereas much right pedal will be required than the entry into an autorotation. So, inadequate right pedal is a common error and the resulting out of trim condition will cause a higher than normal descent rate. Once the needles have split and the rotor RPM has stabilized, the entry is complete.
The Glide: Some instructors/references may use different terminology for this phase of the autorotation, such as “descent phase” or “steady state descent”. From my Army instructor pilot days we used “glide” so that’s the term I continue to use. During the glide portion of the basic autorotation, the potential energy from altitude is being used to create a descent rate or upward airflow through the rotor to maintain the energy in the rotor RPM for use at the bottom. The three primary concerns for the pilot during the glide is attitude (airspeed), trim and rotor RPM. Of these the most important is attitude and note that I said attitude, not airspeed. If the attitude is correct, the airspeed will take care of itself. I wish I had a dollar for every time I’ve seen a pilot who has the aircraft out of trim, causing a decrease on the airspeed indicator, try to correct with forward cyclic. When I’m training someone, many times I’ll cover up the airspeed indicator with my foot or a hand so the entire maneuver has to be done by attitude. (This is especially helpful with the Autorotation with Turn) The attitude should result in the manufacture’s recommended autorotational airspeed ±5 kts. During the glide continually crosscheck attitude, trim and rotor RPM.
The Flare: By the time the flare altitude is reached most of the energy the pilot now has available to decrease the rate of descent in preparation for landing is in the forward speed of the helicopter. Consequently, the flare needs to be well timed and well executed. The flare altitude will, obviously, vary with each type helicopter. Manufacture’s may recommend a flare altitude but this is what I call a “target altitude” because conditions will vary and we’re using outside references to determine the flare point not the altimeter, hence experience (practice) is most important. However, I do think it’s better to error on the high side, so 10 feet to high is better than 10 feet to low. The most important aspect about the flare is not to be mechanical. The rate and amount of flare will vary with wind direction, wind velocity, aircraft weight and density altitude. So, prior to the first autorotation of the day evaluate these variables and adjust the flare accordingly.
At this point I’d like to speak mainly to flight instructors teaching autorotations. As an instructor pilot (IP) in the US Army we used a technique near the end of the glide called “the 100-foot check”. I think so much of this technique I included it, with a few modifications, in Robinson’s Safety Notice No. 38 and the draft I wrote for the US Helicopter Safety Team’s (USHST) Airmanship Bulletin on touchdown autorotations. The mindset on the part of the instructor should be that the autorotation will not continue below 100 feet AGL unless three specific parameters are stabilized: airspeed ±5 kts, rotor RPM in the “green” and a normal rate of descent (add a fourth to the autorotation with turn- “all turns completed”). An excessive rate of descent is an indicator of a number of previous mistakes that I’ll discuss in the autorotation with turn maneuver. If any of the parameters are not where they should be, the instructor should take the controls, bring the power back and terminate the autorotation. Not, take the controls and try to fix this deteriorating situation or talk the student through corrective action–terminate the autorotation. Many of our autorotation accidents are the result of the instructor grabbing the controls below 100 feet to fix an autorotation gone bad and never quite catches up to the helicopter. Don’t try to fix mistakes below 100 feet that were made above 100 feet. Take the controls, terminate the autorotation and go back up to altitude to work on the student’s problems. For operations above 4000 feet density altitude I recommend moving the 100-foot check up to 200 feet.
For instructor training, that is teaching instructors how to teach autorotations, much of the emphasis should be devoted towards being able to make “the 100-foot check” efficiently enough to prevent the helicopter from descending below 100 feet in autorotation if things aren’t perfect. It takes a bit of practice to determine where in the glide to begin making the 100-foot check, then make the go-no-go decision to continue or not and, if necessary, then take the controls all before the aircraft descends below 100 feet.
One last comment to instructors, when you are on downwind for a practice autorotation, just remember, you are about to perform the number one maneuver that causes accidents in helicopter training.
The Power Recovery: This discussion will only deal with a power recovery for the termination of the practice autorotation. Those interested in touchdown autos, please see the essay on “Touchdown Autorotations”. The purpose of the power recovery is to restore engine power in order to terminate the autorotation at a stabilized three, five or eight foot hover depending on the helicopter. The key word here is “stabilized” meaning zero vertical descent and little or no forward/ lateral movement. The power recovery in a piston powered helicopter is quite different from turbine so let’s talk about each individually.
The piston helicopter is fairly straight forward, if you want power increase the throttle, join the needles then raise the collective. Power comes back almost instantaneously (This may not be the case with turbocharged piston engines, in which case the turbine engine discussion below may be more appropriate). Therefore you can delay starting the power recovery until the effects of the flare are almost completely dissipated and the descent is becoming more vertical. I like to begin bringing the power back in by increasing the collective while the helicopter is being leveled, but with the nose still slightly high. This way the collective increase stops, not only descent rate but also forward movement, shortening the ground run. I use the same technique on a touchdown autorotation. One of the most common autorotation accident scenarios (even in power recovery autos) is holding the flare too long trying to recover every bit of energy possible and sticking the tail rotor in the ground. If the flare was too little or too low, take your medicine and accept a higher forward speed. Don’t try to correct a mistake at 10 feet that was made at 40-50 feet. Due to this relatively fast response time of the piston engine the power recovery maneuver simulates the real thing very closely so I think there is a great deal of value in the power recovery without all the risks associated with going to the ground.
The turbine engine presents a whole set of additional problems which greatly detracts from the training value of a power recovery. There is an inherent lag in the turbine from when the pilot asks for power and when the engine delivers the power especially when the RPM is low which can cause an over torque/temp. Because of this lag, it’s best to join the needles to begin the power recovery just prior to, or at the beginning, of the flare. That way the turbine has time to speed up during the flare so when the pilot asks for power at the end of the flare, it’s there. Consequently, the flare and recovery are, in my opinion, not realistic. I remember when the US Army switched from always doing autorotations to the ground to never doing them to the ground outside of initial pilot training (late 1980s). All the IP’s (instructor pilots) hated it. When I fly an R66 at the Robinson Safety Course, I feel an obligation to teach the power recovery and then it’s quickly on to touchdowns. However, having said that, in turbine helicopters with lower inertia rotor systems, operations at high gross weights or high density altitudes the power recovery may be the best option.
The Autorotation with Turn
The purpose of the autorotation with turn is to teach or practice turning the helicopter during the glide portion of an autorotative descent. As we will see with the Power Failure From Altitude maneuver, turns, or a series of turns, are one of the primary tools the pilot has to maneuver the helicopter to the point of intended landing after an engine failure. For training I think we should practice autorotative turns beginning at 90º and working up to 180º. I am a strong believer in the “progressive approach” to teaching autorotations and this is especially true with the autorotation with turn. For those who might be unfamiliar with the “progressive approach” refer to FAA Advisory Circular (AC) -140A “Autorotation Training”.
The entry into an autorotation with turn is the same as the basic autorotation with one modification. The entry altitude should be higher, a minimum of 700 ft AGL especially if the turn will be closer to 180º. But this is the minimum, initially the instructor should go up to 1000 ft or perhaps even higher and as student proficiency increases we can reduce the altitude. Sure, a 180º turn can be done from 500 ft AGL and with a good pilot, even lower. However, the risk is much higher for a reward with little place in the real world.
30% of all Robinson accidents are in autorotation and 75% of all these accidents are doing 180s. The major concern in the 180 or autorotation with turn is allowing an excessive descent rate to build in the turn then as the helicopter gets closer to the ground the pilot and/or the instructor may lose the ability (altitude) to dissipate this high descent rate. There are four common errors that cause high descent rates when turning in an autorotation and the instructor needs to have their antenna out looking for one, or a combination of these four. They are: out of trim, nose low, high rotor RPM and high bank angles.
-
Out of trim–The entry into the autorotation requires a large right pedal input, perhaps the largest right pedal input of any maneuver. Consequently, it is not uncommon for the pilot to apply too little or too much right pedal. The out of trim condition increases the parasitic drag thus increasing the descent rate. Additionally, during a turn it is not uncommon for the pilot to use pedal in the turn's direction causing an out of trim condition.
-
High rotor RPM–Rotor RPM in the higher ranges of the allowable autorotational RPM cause higher descent rates. As much as 500-700 fpm difference can exist between the lowest allowable RPM and the highest. High aircraft weights can also cause the rotor RPM to stabilize in the higher RPM ranges. When in a dual configuration (instructor & student) most small training helicopters are flown at the high end of the weight regime resulting in higher Rotor RPMs and increased descent rates. Turns will also increase the rotor RPM because of the increase in G loading caused by the increased load factor.
-
Nose low/high airspeed–An excessive nose low attitude causing the aircraft to dive will also increase descent rates. Aircraft attitude, especially during a turn, is perhaps the most important element to control.
-
High bank angles during a turn–High bank angles will also increase the descent rate. In training, bank angles should be limited to 40° or less. Commonly high bank angles will occur in conjunction with a nose low attitude increasing descent rates.
As I mentioned, frequently a combination of these errors occur during the same autorotation. From the instructor’s standpoint if two or more of these errors occur I would not even wait for the high descent rate to develop, just take the controls and terminate the maneuver.
The Advanced Autorotation
The Advanced Autorotation is the same as what SFAR 73 (Special Federal Aviation Regulation) calls ‘Enhanced Autorotations”. During discussions within the ACS Working Group, I pushed to adopt the SFAR terminology for this maneuver so there would not be different terms for the same maneuver, but the group felt the word “advanced” fit better with the term “basic autorotation”. Since the two are the same, refer to the discussion below.
Enhanced Autorotation Procedures
I had never heard the term “Enhanced autorotation procedures” until Special FAR 73 was published in 1995 (paragraph 2(b)(1)(ii)). Actually, the SFAR calls the training “Enhanced training in autorotation procedures” but at Robinson we’ve shortened it too just “Enhanced autorotation procedures”. So, I asked Bob O’Haver who was in charge of the FAA’s Flight Evaluation Board (FEB) that recommended the SFAR and was the rule’s author, what he meant. He said “Tim, when you have the occasion to write a new rule, if there is something that’s always bothered you, it’s an opportunity for you to address it.” He went on to tell me that he had always been disappointed in the autorotation training pilots, especially private pilots, received. Autorotations were always practiced at the same location, from the same traffic pattern, at the same altitudes and airspeeds. He wanted instructors and schools to vary these elements and I couldn’t have agreed more. However, poor autorotation training or execution had nothing to do in any way with the purpose of Special FAR 73. He put enhanced autorotation training into the new rule and in the FAA’s rush to judgement to get the rule published (The FAA conceived the rule, wrote it, staffed it and published it in 30 days.) it never came out. To this day there is still no formal FAA definition “enhanced autorotations”, so I guess, it’s whatever a school or an instructor wants it to be. At Robinson we have developed a comprehensive training program around the concept of enhanced autorotations which we define as an “understanding and application of different elements and procedures used to safely maneuver the helicopter to a specific spot in autorotation”.
I think it’s most important when the real-world engine failure occurs that the pilot can maneuver the aircraft to the point of intended landing with enough airspeed for a flare, enough rotor RPM for a landing and without an excessive rate of descent. It’s of no use at all to perform a picture-perfect autorotation and end up 30 feet short in the trees. Remember, it doesn’t have to be pretty to be successful. There are quite a few, what I’m going to call “tools”, the pilot has available to “make the spot”. I will discuss each one individually but one or more can and maybe should be, combined in one autorotative glide.
Area selection:
When the engine quits and all the cockpit tasks are complete in transitioning to an autorotative glide, the first thing the pilot needs to do is select a landing area. Have a selection plan in mind, for example start at 9/10 o’clock and scan to 2/3 o’clock or do the reverse, perhaps look first into the wind then scan from there until a suitable site is found, but move your head. I see it over and over on a private and even commercial pilot check ride where I set the helicopter up with a large landing area 90º to the left or right, reduce the throttle to simulate the engine failure and the pilot never moves the head and picks some postage stamp size parking lot straight ahead.
Once the landing area is selected, the first question to ask is “Am I going to be long or short?” (It’s your lucky day if there’s a big flat clear area right in front of you and the traffic pattern autorotation is all you need.) The way you tell is the same as an approach: if the spot moves up on the windshield your short, if the spot moves down you’re long. If the glide will be short go to maximum glide configuration, which we’ll discuss in a moment. If the spot moves down on the windshield indicating the glide will be long, now is where the enhanced autorotation procedures come into play. I’m going to use the “Enhanced Autorotation Procedures” section from the R44 Maneuver Guide that I originally wrote in 2010 but have updated to be more generic for this discussion.
There are four rules I recommend for enhanced autorotations, especially during training because it’s just that – training. They are:
-
At 500 ft AGL all tools involving decreased airspeed should be completed and the nose lowered to accelerate back to the normal autorotative airspeed.
-
Below 500 ft AGL if the glide still needs to be reduced, “S” turns are the only tool to use.
-
Never make a turn so sight of the landing area is lost.
-
Always have an instructor experienced with these tools at one set of the controls.
Use of turns:
Left, right or “S” turns can be used to decrease the glide distance in autorotation and this is probably the most common tool. It is important to maintain proper attitude and trim when turning to prevent increased descent rates and adjust the collective as necessary in the turn to maintain rotor RPM. A large turn (270°-360°) is not recommended since for much of the turn the pilot will lose sight of the landing area and will be unable to determine the effect of wind on the glide, perhaps leaving the helicopter short once the spot is back in view. Multiple “S” turns at the normal autorotative airspeed can also be a difficult maneuver for pilots because of the need to maintain attitude and trim while banking back and forth. Use of airspeed is a much better alternative in these cases.
Use of airspeed:
Airspeeds from zero to maximum glide airspeed can be used to adjust the autorotative glide distance as necessary. Maximum glide distance configuration is covered later so this discussion concerns reducing airspeed. I recommend an entry altitude of 1500 ft to 2000 ft AGL be used so the student/pilot can get an extended time flying at the lower speeds in autorotation. Enter the autorotation so that a normal glide will take the helicopter well past the point of intended landing thus requiring a shortened glide distance. Once the glide is established apply aft cyclic to a 10°-15° nose high attitude. Adjust the collective as necessary to control increases in rotor RPM. At approximately 25–30 kts (the airspeed indicator accuracy is problematical at this point) the nose of the aircraft will come down to a level attitude on its own due to the upward flow of air acting on the tail cone and horizontal stabilizer. Maintain this configuration and observe the landing area moving up on the windshield. Expect the descent rate to increase, however, the amount will vary with type helicopter, wind and weight. At this point the landing area will be right in front providing an excellent opportunity to continue to survey the condition of the landing surface. When a normal Autorotative angle is achieved or approaching 500 ft AGL, lower the nose to 10°-15° nose down to increase airspeed back to the normal autorotative speed.
Use of pedals:
With this tool the pedals will be used to turn the helicopter instead of banking with the cyclic. Set the maneuver up at 1500-2000 ft AGL with the point of intended landing and the wind 90° from the helicopter’s ground track (out the left or right door). Many pilots in this situation will attempt a 270º turn (left or right as appropriate) but for a large portion of the turn the landing spot will not be in view and the opportunity to further recon the landing area is lost. Since the left pedal is the strong pedal in autorotation, I recommend the landing area be positioned out the left door requiring left pedal to turn. However, the student/pilot should experience use of both pedals. Enter the autorotation and slow the airspeed as in the “use of airspeed” maneuver. Once the nose comes down to the level attitude, apply pedal to align the aircraft with the desired track towards the landing area. Use of left pedal will decrease the rotor RPM requiring the pilot to lower the collective as necessary to maintain the rotor RPM. The remainder of the maneuver is the same as the “use of airspeed” discussion.
Use of sideward flight
The purpose of using sideward flight in an autorotative glide is when the point of intended landing is almost directly below the helicopter. Set up the condition with the wind 90° (left or right) from the aircraft’s ground track at 1500 FT-2000 ft. Enter the autorotation directly over or just prior to the intended landing area. Decrease the airspeed as in the “use of airspeed” maneuver. When the nose comes down to the level attitude apply lateral cyclic in the direction of the desired sideward flight and opposite pedal to prevent the aircraft from weather vaneing towards the direction of flight. The resulting slip and wind direction will move the helicopter downwind from the landing area. Once the helicopter is far enough away from the landing area stop the sideward flight with opposite cyclic. Opposite pedal is then applied (as in the “use of pedal” maneuver) to align the aircraft into the wind tracking towards the landing area and lower the nose to regain airspeed. Again, left pedal is the strong pedal in autorotation, so sideward flight to the right, requiring the use of left pedal is the easier of the two choices.
Maximum glide distance configuration:
In 14 CFR § 27 approved helicopters the maximum glide distance configuration is found in the Pilot’s Operating Handbook (POH) or Rotorcraft Flight manual (RFM). For this discussion I am going to use the Robinson R44 maximum glide configuration of 90kts at 90% rotor RPM. The purpose in practicing this maneuver is not only to glide the furthest distance in autorotation but also, to get the pilot accustomed to the low RPM warning system being on for extended periods and developing the pilot’s ability to determine if a glide will be “too long” or “too short”. Enter the autorotation at 1500ft-2000ft to give the student an extended time to fly at the max glide configuration. Adjust the airspeed to 90kts and increase the collective to set the rotor RPM at 90% (The low RPM horn/light will be on the entire time the RPM is below 95%.). It’s very important to keep the aircraft in trim during the entire glide. While at the max glide configuration reference any movement of the point of intended landing up or down on the windshield. If the landing area moves up the glide will not reach the area and a new area should be selected. Eventually the landing area may begin to move down indicating the glide will take the aircraft past the area so exit the max glide configuration. Allow the rotor RPM to build back into the normal range below 500ft.
There is a trick that can be used below 500ft AGL to squeeze additional 200 to 300 feet and sometimes even more out of the max glide (depends on wind, weight and the helicopter type). Maintain the max glide configuration down to approximately 100ft AGL then slowly decelerate with aft cyclic (careful not to balloon) and coordinate with lowering collective. The energy from the high airspeed is being used to increase the glide distance and rotor RPM. Begin the flare at the normal flare airspeed and altitude.
Minimum rate of descent configuration:
The minimum rate of descent configuration is used to gain more time (rate), not distance, time. For example, you might need more time in an autorotation to try an air restart, make a mayday call, finish your prayers or maybe just put off the inevitable. Minimum rate of descent configuration may or may not be in the POH or RFM depending on when the model was certified. It is in the R44 POH but not in the R22’s. However, it’s easily determined. For all helicopters the minimum rate of descent configuration will always be best rate of climb speed (Vy) at the lowest allowable rotor RPM for autorotation. Again, I’m going to use the R44 minimum rate of descent configuration in my discussion which is 55kts at 90% rotor RPM. The purpose of practicing this maneuver is not only to autorotate at the slowest descent rate but also, to practice preforming another procedure such as an air restart or mayday call while still flying the helicopter in autorotation. Enter the autorotation at 1500ft-2000ft. After establishing an autorotative glide simultaneously apply aft cyclic to slow the helicopter to the appropriate airspeed and increase the collective to bring the rotor RPM down to 90%. Realize that these two control inputs compete with each other, in that the aft cyclic tends to increase the RPM while the pilot is trying to reduce the RPM with the collective. First, build proficiency establishing and maintaining the minimum rate of descent configuration then add preforming a simulated air restart or mayday call into the autorotation. Return to the normal airspeed and rotor RPM prior to descending below 500ft AGL. An interesting sidelight to this maneuver is to demonstrate the effect of rotor RPM on descent rate. Once established at the minimum rate of descent configuration and the descent rate has stabilized, lower the collective full down (maintain attitude) then observe the descent rate increase, especially at higher weights. In an R22 at maximum gross weight there is a significant difference of almost a 500 foot/minute between the lowest allowable RPM (90%) and the highest (110%).
Backward or rearward flight:
I’ve saved this discussion for the last because I only recommend this in very specific situations by very experienced pilots. This maneuver can develop extremely high descent rates that the pilot must anticipate during the transition to normal autorotative flight. I would only use rearward flight if I had a 25+ kt headwind and my landing area was immediately below me or slightly behind me. When demonstrating this technique I use the scenario of having to land on a very small island surrounded by sharks. In this case any turn downwind followed by a turn back into the wind will be very difficult to judge. Decelerate as in the “use of airspeed” maneuver but this time don’t let the nose drop to a level attitude, maintain the nose high attitude. As the aircraft begins to move backwards in the autorotation (note groundtrack) the pedals become very sensitive and large yaw rates can develop (I think they’re confused as to what’s going on) so quickly correct heading changes with the pedals.(If you’re in a turbine you’ll begin detect that wonderful smell of burning jet fuel.) Once the landing area moves out in front of the helicopter lower the nose to a significant nose low attitude (20˚ or more) to transition to normal autorotative flight. This is the point where in light wind conditions the descent rate will get quite high requiring substantial altitude to dissipate it. Whereas, in a very strong wind the helicopter is actually being blown backwards by the wind and the transition is much easier.
Putting it all together:
After practicing the above techniques and developing a degree of proficiency with each tool, it’s time to put all the tools into the toolbox and work on determining which tools to use as part of a plan to “make the spot”. If after selecting the landing area the answer to that first question is you’re going to be long the next thing is to develop a mental plan as to what tools to use then when and how to use them. Ask yourself; Do I have to get further away from the area? Do I have to maneuver into the wind? Are there obstacles/barriers around the landing area? Part of the plan should include how to keep the spot in view at all times, perhaps with the pedals or sideward flight. I find it a little easier to stay in relatively close to the area as altitude is being lost because there are four tools to use (turns, airspeed, pedals and sideward flight) whereas if you get to far away there is only max glide configuration. Another important consideration in training is it’s not really necessary to take the maneuver all the way down to a flare and power recovery. Remember, the whole purpose is to learn how to maneuver the helicopter to a specific spot and in many instances this can be determined long before the flare. Terminating the autorotation and going around before the flare is certainly safer without compromising the training.
I want to make one last comment about enhanced autorotation training. I am amazed at the confidence pilots feel in their ability to put the helicopter where they need to in an autorotation after some extensive training in these techniques. A few years ago I was teaching a Safety Course about 50 miles outside of Moscow where the terrain is flat but a dense forest of 80 foot trees stretches in all directions as far as the eye could see. Small, intermittent clearings large enough to hold a couple of helicopters dotted the landscape. After a few hours of training the pilots stopped talking about the best way to autorotate into the trees and now confidently plan to “make the spot”.
Touchdown Autorotations
In 2013 the US Helicopter Safety Team (USHST) suggested the FAA publish an Advisory Circular (AC) on “Touchdown Autorotations”. The USHST Training Working Group (TWG) was tasked to prepare a draft that the FAA could then work from. I wrote the draft for the Training Working Group which was then peer reviewed by all the group’s members and suggestions were incorporated into the draft. In April 2013 the USHST Executive Committee reviewed and approved the draft then forwarded it to the FAA hoping a new AC would result. The FAA expanded the draft considerably to encompass several other emergency procedures, added other techniques and, to be honest, created a confusing new document. The USHST rescinded its recommendation and the FAA never published the AC. However, the USHST took their draft and published the following four page “Airmanship Bulletin” which I will use parts of in several essays concerning aspects of the autorotation.
USHST Airmanship Bulletin:
Touchdown Autorotations
Purpose:
The purpose of this Airmanship Bulletin is to provide specific techniques and procedures to enhance touchdown autorotation training and safety.
Objective:
The objective is to reduce the likelihood of an accident during touchdown autorotation training. For the sake of discussion this bulletin assumes a helicopter with the rotor turning counterclockwise as viewed from the top.
Background:
The value of touchdown autorotation training has been the subject of constant debate within the helicopter community for many years. One side argues the benefit the pilot receives in actually experiencing an autorotative landing and the opportunity to build proficiency in the technique greatly increases pilot confidence, thus reducing the chance of a catastrophic outcome to a real engine failure. They believe the power recovery aspect of the autorotation does not resemble the real situation and may even build a false sense of security on the part of the pilot. The other side claims the increased risk of damaging the aircraft with the touchdown maneuver is not worth the benefit gained over terminating with power at a hover. They feel that with the increased reliability of today’s modern engines, the helicopter community will damage/crash more aircraft practicing for an event that rarely occurs. This Airmanship Bulletin does not take either side but does acknowledge the controversy continues to exist.
The FAA Practical Test Standards do not require the applicant to demonstrate proficiency at touchdown autorotations during the private, commercial or ATP practical test. Instead, preferring a power recovery as appropriate. However, the Flight Instructor Practical Test Standards do require a CFI applicant to demonstrate proficiency in touchdown autorotations. The thinking being the flight instructor, who is frequently splitting the needles with practice autorotations, is much more at risk to actually have to perform a real autorotation. FAR Part 135 initial and recurrent training, also, does not require a touchdown autorotation. The FAA does conduct touchdown autorotation training for FAA inspectors during their helicopter recurrent training using the same logic as with the flight instructors. Most law enforcement agencies and many commercial operators require touchdown autorotations during recurrent training but it’s a very common practice to contract out this training rather than perform the maneuver in their own aircraft. Throughout the 1960’s, 70’s and most of the 80’s the US Army conducted only touchdown autorotation training for both initial aviator qualification and yearly recurrent training. However, in the late 1980’s the Army eliminated touchdown autorotations for all but initial training due to an excessively high accident rate.
Teaching touchdown autorotations requires a high degree of experience and proficiency on the part of the instructor. It is recommended these autorotations be practiced only with an instructor experienced in the particular make and model and current in performing touchdown autorotations.
Environmental/Aircraft conditions:
Due to the critical nature of the touchdown maneuver all environmental conditions and variables the pilot can control should be used to create the safest conditions in which to conduct the training. It is recommended that wind velocity be at least 5 knots and no more than 30° from the final approach heading. The stronger the headwind, the more energy available and the larger the margin for error. Aircraft weight is another large variable. The lower the aircraft weight the better the relationship between the amount of energy available versus the amount of energy needed for a safe touchdown maneuver. Flight instructors should adjust aircraft weight as low as possible by reducing fuel loads, prohibiting passengers and removing certain mission equipment (searchlights, cameras, etc.) when able. Once proficiency at lower weights is developed or for advanced training, organizations may want to increase aircraft weights closer to a normal mission profile. Wind and weight requirements should be adjusted when practicing touchdown autorotation at higher density altitudes (above 4000 feet). During the preflight insure the aircraft’s skid gear, skid tubes and skid shoes are in good condition. It is recommended that touchdown autorotations be practiced to hard surfaces such as runways or taxiways. This allows the skid gear to slide on the hard surface if the landing is made with any sideward movement reducing the possibility of a rollover. The tradeoff is when landing with very high descent rates. The hard surface is not as forgiving as softer grass or sod surfaces. However, as long as the landing is made with the skids level, the skid gear will yield, as it’s designed to do, absorbing the energy of a hard landing. Touchdown autorotations require a high degree of concentration on the part of both student and instructor. Therefore, it is recommended only 3-5 touchdowns should be performed then take a short break doing easier maneuvers before returning to the touchdowns.
Preparatory maneuvers:
Prior to the actual touchdown autorotation a number of preparatory maneuvers can be practiced to help gain an understanding of what the actual touchdown maneuver will be like. This is especially helpful for students just beginning their touchdown autorotation training and pilots going through recurrent training in which the touchdown maneuver has not been practiced for an extended period of time. Power on running landings will help prepare the student for landing in the autorotation with forward speed. Hovering autorotations from both a stabilized hover and from a hover taxi simulate the final stages of the touchdown autorotation. Finally, two or three power recovery autorotations should be practiced to properly gauge the effects of wind and aircraft weight on the autorotation technique for that particular day.
Four phases of the autorotation:
The four phases of the autorotation are the entry, the glide/turn, the flare and the touchdown. Elements of the entry and glide/turn are the same as in the practice autorotation with a power recovery while the flare and touchdown phases, although similar, have unique elements. Downwind checks, final checks, entry airspeeds and altitudes will be the same.
The Entry: The purpose of the entry in any practice autorotation is to teach or practice the elements of an autorotational glide/turn, flare and recovery/landing, not how to recognize and properly react to an engine failure (This should be done with the simulated engine failure/forced landing maneuver). Therefore, the entry into the autorotation should be accomplished as easily and smoothly as possible. A hurried, improper entry can create a very high pilot workload during the remainder of the autorotation. Smoothly but firmly lower the collective full down while simultaneously adding right pedal to maintain the aircraft in trim. When the collective is lowered during the entry the aircraft’s nose will pitch down due to dissymmetry of lift, flapping and gyroscopic precession, regardless of airspeed (the reverse of blow back or flap back). This will increase the rotor RPM decay rate and, if not corrected soon enough, the nose low attitude will also increase the descent rate. Consequently, aft cyclic should be used during the decrease of collective to maintain the entry attitude. Once the airflow through the rotor has reversed and an autorotational glide has been established adjustments to aircraft attitude can be made. When the collective is full down retard the throttle to split the needles. In some turbine-powered aircraft it may be preferable to retard the throttle prior to lowering the collective to prevent overspeeding of the RPM. Once the collective is full down, cross check attitude, rotor RPM, trim and airspeed.
The Glide/Turn: During the glide portion of the autorotation maintain the aircraft in trim, recommended airspeed +/- 5 knots and rotor RPM in the green range. At some point during the glide the decision must be made to fully close the throttle. This decision might vary with different instructors and different aircraft but should be accomplished at a reasonable height after the point where a landing at the intended touchdown area is assured. Avoiding high descent rates, especially during turns is critical during the glide phase. There are four common errors can cause excessive descent rates during the autorotation. The instructor should focus on detecting one or, in many instances, a combination of these elements.
-
Out of trim – During the entry into the autorotation a large right pedal input is required, perhaps the largest right pedal input of any maneuver. Consequently, it is not uncommon for the student to apply too little or too much right pedal. The out of trim condition increases the parasitic drag thus increasing the descent rate. Additionally, during a turn it is not uncommon for the student to use pedal in the direction of the turn causing an out of trim condition.
-
High rotor RPM – Rotor RPM in the higher ranges of the allowable autorotational RPM cause higher descent rates. As much as 500-700 fpm differences can exists between the lowest allowable RPM and the highest. High aircraft weights can also cause the rotor RPM to stabilize in the higher RPM ranges. When in a dual configuration (instructor & student) most small training helicopters are operated towards the high end of the weight regime resulting in higher Rotor RPMs and increased descent rates. Turns will also increase the rotor RPM due to the increase in G loading caused by the increased load factor.
-
Nose low/high airspeed – An excessive nose low attitude causing the aircraft to dive will also increase descent rates. Aircraft attitude, especially during a turn, is perhaps the most important element to control.
4. High bank angles during a turn – High bank angles will also increase the descent rate. In training, bank angles should be limited to 40° or less. Quite commonly high bank angles will occur in conjunction with a nose low attitude greatly increasing descent rates.
The 100 ft Decision Check:
A very valuable technique developed by the US Army is the “100 ft decision check””. The idea is to create a decision point at which the instructor, based on specific parameters, makes a decision to either continue the autorotation or return to powered flight. The mindset of the instructor should be to not allow the aircraft to descend below 100 ft AGL in autorotation unless four parameters are met:
-
Airspeed/attitude +/- 5 kts
-
Rotor RPM in the green range
-
A normal rate of descent (rates vary with model helicopter)
-
All turns completed and proper alignment
If any of these parameters are not met, the instructor must take the controls, reintroduce the power and commence a go around. Instructors should not talk the student through corrective action or take the controls and attempt to fix the autorotation. Go Around! Students should be thoroughly briefed on the aspects of this check and well aware of the possible outcomes. Instructor training should develop the discipline and technique to initiate then react to the results of a timely 100 ft check prior to descending below 100 ft. Instructors may want to increase the decision check to 200 feet AGL based on high density altitudes (above 4000 ft), specific aircraft flare altitudes and power recovery techniques.
The Flare: The purpose of the flare is to decrease both rate of descent and ground speed. The flare is the most critical phase of the touchdown autorotation because most of the energy available to the pilot in autorotation is in the flare. Consequently, the flare must be well timed and correctly executed. Flight instructors must focus intently on the effects of the flare and revert to a power recovery at the earliest sign of a poorly executed flare. The altitude the flare is initiated varies with aircraft type, wind and gross weight but, as a general rule, the flare starts at an altitude approximately 1 ½ times the diameter of the main rotor and can be found in each aircraft Pilot Operating Handbook (POH). Erring a little on the high side is probably preferable to erroring a little on the low side. Too much flare causes the aircraft to “balloon” risking development of a high descent rate and too little flare does not adequately reduce the ground speed causing high touchdown speeds.
The Touchdown: The touchdown phase of the autorotation varies greatly between helicopter make and models. This is why instructors need to be proficient and current in the make and model in which the autorotation is being performed. By the touchdown phase all the energy left for the landing is in the rotor RPM. Consequently, the collective increase needs to be well timed for the conditions. Unlike the power recovery autorotation, the nose will yaw to the left when the collective is increased due to the friction through the main rotor gearbox and drive train. This need for right pedal may be contrary to the normal habit pattern of a pilot new to the touchdown phase. Arguably, the most important aspect of the touchdown is to land in a level flight attitude with the skids parallel to the ground track. Landing with the aircraft drifting left or right, skids offset from the ground track or excessively on the “heels” or “toes” of the skid gear can all result in aircraft accidents. Once the aircraft is on the ground the collective can be slowly lowered to act as a brake (assuming the landing is on a hard surface). Aft cyclic should not be used as excessive blade flapping could lead to tail boom contact.
Turbine vs Piston Engines:
There are a number of differences between the turbine engine and the piston engine affecting the autorotation that instructors/pilots should be aware of. The most important is the lag in response time between the two. The piston engine will respond very quickly if the pilot decides to revert to a power recovery, however the turbine engine is very slow to respond. This slow response rate of the turbine engine requires the pilot to make the decision to proceed with the touchdown or make a power recovery much earlier (In some cases before the flare.) than in the piston engine. Additionally, because of this slow response rate, power has to be brought back in at a much slower pace in the turbine. Pilots should also understand that when the turbine engine is reduced to idle the fuel control deceleration schedule causes the torque to decrease gradually. Due to this additional output torque the left yaw and rotor decay rate is much more docile on the practice auto than if the engine actually flamed out. This difference can be significant however, most organizations, including the FAA, understand and accept this reality, as the alternative is not very attractive from a safety standpoint.
Conclusions:
Although the value of touchdown autorotation training is still debated there are instances where it is required and justified. Operators, instructors and pilots need to insure that the procedures and techniques discussed in this Airmanship Bulletin are used to conduct this training in the safest possible manner. Instructor experience, currency, aircraft characteristics and environmental conditions must be assessed and adjusted for each training flight.
The Height-Velocity (H-V) Diagram
When I first got involved with the construction of an H-V diagram (1983 with Robinson test pilot Terry Strange in the R22 Alpha) it amazed me how little I knew about a helicopter’s height-velocity diagram. I’d been an FAA instructor for ten years, had been through the US Army’s UH-1 Instructor Pilot course and all I knew was “stay out of the shaded area”. That’s about all most manufactures, including Robinson, tell the pilot. Nothing about the phases of flight (takeoff, cruise etc.), control input delays, flight techniques used, or variables that make the curve get larger or smaller – just “stay out of the shaded area”. I think you’ll see there’s a lot more going on in this chart than just “stay out of the shaded area”.
The purpose of the height-velocity diagram is to indicate combinations of airspeed and height above the ground which will allow the average pilot to successfully complete a landing after an engine failure. A couple of interesting words in that statement. What is an “average” pilot and what is a “successful” landing after an engine failure? Let’s take the average pilot first. Do you think the manufacture’s test pilot, any manufacture’s test pilot, who determines points along the H-V curve can be successfully accomplished qualifies as “the average pilot”, probably not. Then, if this above average pilot has to make a subjective determination that the average pilot could also complete this autorotation, do you think he might possibility be influenced by that bank deposit he receives from his boss every two weeks - sure. So, the manufacture’s test pilot will demonstrate that points around the curve can be successfully performed, then an FAA test pilot will duplicate these points and, at the end of the day, it will be the FAA test pilot that makes this subjective determination whether an average pilot could also complete the landing. So, the first bubble I want to break is that points along the curve do not represent combinations where the helicopter can or cannot complete a successful autorotation, rather, where someone determined the “average pilot” could be successful. Now, as far as what is a “successful landing after an engine failure”, I’ll bet if I asked three people what they would consider a successful landing if the engine quit on their next cross country, I might get three different answers. Some might be satisfied with just walking away while others might even be happy with crawling away. As far as this test goes, after the helicopter comes to a complete stop, if there is any component that has to be replaced it is an unsuccessful landing. The autorotations don’t have to be pretty but you can’t bend or break anything.
TEST CONDITIONS
Current regulations regarding the construction of a height-velocity diagram are located in 14 CFR § 27 Airworthiness Standards: Normal Category Rotorcraft (maximum weight 7000 pounds or less and nine or less passenger seats), where it is called a “limiting height-speed envelope”. There are two parameters that § 27.87 establishes for the test – altitude and weight. A sea-level curve is developed at maximum gross weight then a 7000-foot density altitude curve is also developed. However, the high-altitude H-V can be conducted at a lower weight selected by the manufacturer, but not less than the maximum weight to hover OGE. This high-altitude H-V diagram is new to Part 27 and you won’t find one in flight manuals for aircraft certified under the older rules (Civil Aviation Regulation 6), such as the Jet Ranger or the MD 500. Other requirements include calm wind (2 kts or less), minimum crew, minimum instrument panel (although a radar altimeter and a more accurately calibrated airspeed indicator are allowed in the interest of accuracy) and if there is a more critical CG, then the test has to be conducted at that CG (not applicable for any Robinson Helicopter). Demonstrated conditions such as “smooth hard surface” and “governor on” are also listed on all Robinson H-V diagrams. Obviously, the engine failure is simulated by rolling the throttle off to split the needles not by actually shutting the engine down. This is straightforward with a piston engine but with a turbine engine there will still be output torque from the N1 deceleration schedule set in the fuel control unit. Part 27 accepts this additional horsepower as the only alternative would be to flame the engine out which, of course, increases the risk substantially. However, after the R66 H-V test was completed by rolling the throttle back to idle, one point (the knee) was accomplished with the engine shutdown. The rotor decay difference is noticeable but not significant.
H-V TERMS
Using the R44 Raven I chart below as my example I’ll go around the diagram and explain the requirements for each point or area.
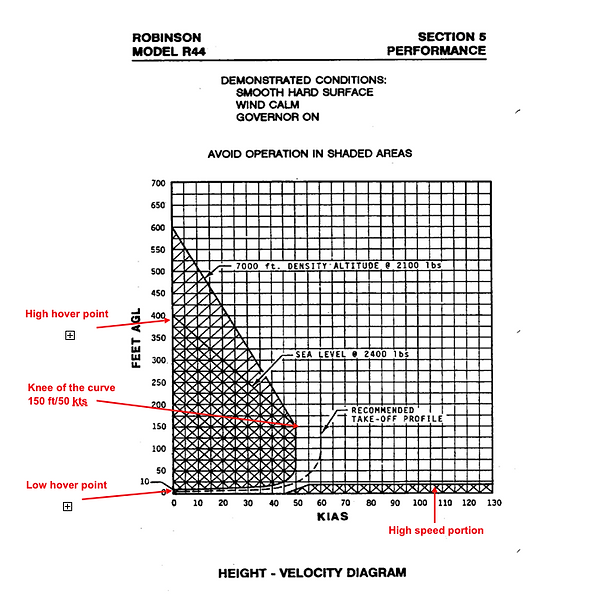
The Low Hover Point – 10 ft/0 kts
-
No flare to gain any airspeed is allowed. The aircraft must come straight down.
-
The collective cannot be lowered. Although this may be a common pilot technique for higher hovering autorotations, it is not permitted because it is not a pilot action which could be expected if the engine fails without notice during a hover.
-
The autorotation must be done on a hard and soft surface
The High Hover Point – 400 ft/0 kts
-
Aircraft must be in a stabilized OGE hover with little vertical movement. Use of a radar altimeter is essential for this point.
-
A 1 second delay before lowering the collective is required which will obviously cause a high rotor RPM decay rate due to hovering OGE at maximum gross weight.
-
After the delay and the collective is lowered, the technique is to lower the nose using the available altitude to increase speed, and then using the energy from the speed to make a successful landing. The FAA does not think it reasonable for the average pilot to put the aircraft in an excessive nose down attitude to gain speed so a dive angle of 10∞ to 20∞ is recommended.
The “Knee” of the Curve – 150 ft/50 kts
-
The knee separates the level flight or cruise portion from the takeoff portion.
The Level Flight or Cruise Portion
-
Extends from the high hover point down to the knee of the curve.
-
Power as required to maintain level flight.
-
A 1 second delay before lowering the collective is required.
The Takeoff Portion
-
Takeoff power (R22/R44) or a power selected by the manufacturer as an operating procedure (hover power plus 5% torque for the R66) must be used.
-
There is no required delay on lowering the collective, rather, what is called “normal pilot reaction time” is used.
-
Unlike the low hover point, the collective may be lowered to save rotor energy then raised prior to ground contact.
-
A cyclic flare to use energy from any forward airspeed is acceptable.
-
Considerable ground run is not only acceptable but is normal.
I feel the takeoff portion of the H-V diagram is by far and away the most critical portion because of the combination of maximum power being used, hence a faster rotor RPM decay and the fact the helicopter is climbing. When the engine fails, the climb must first be dissipated before the aircraft descends allowing the aerodynamics forces from the upward airflow to drive the rotor. For example, if the helicopter was climbing at 1000 ft/min when the engine failed, rotor RPM is decaying the entire time that 1000 ft/min climb rate is being dissipated. Consequently, I don’t recommend any practice autorotations at the higher end of the takeoff portion, even if a power recovery is intended, because if the engine fails, you have a tiger by the tail. If you must practice engine failures on takeoff, accelerate out to the 60-70 kt range where some margins exist if the engine actually quits. Also, I think it’s important for pilots to realize this portion is based on takeoff and the conditions that make the takeoff so critical don’t exist on an approach. On an approach the helicopter is already descending and the power is relatively low so the time it takes for the aerodynamic forces to take over are quicker and the rotor decay rate slower. I still find pilots who maintain a high speed throughout a normal approach and when asked why? The response is something like “I’m trying to stay out of the shaded area of the H-V diagram”. They are mixing apples and oranges – trying to stay out of an area on an approach that was constructed for takeoff. So, nice slow approaches.
The Takeoff Corridor
Part 27 requires the manufacturer to depict the Takeoff Corridor, which Robinson calls the “Recommended Takeoff Profile”. Since this was not required under the old CAR 6 rules, it’s one of the best ways to determine if an H-V diagram was constructed under the old or new rules. The Takeoff Corridor has to be wide enough to permit a takeoff path clear of the shaded area using “normal pilot skill”.
The High Speed Portion
-
Normal pilot reaction time to lower the collective.
-
Power as required for level flight
If we calculated the amount of kinetic energy available at the high airspeeds in this portion (see my essay “Energy Management in Autorotations”), we’d see there is more than enough energy to make a successful landing. In fact, much of the energy has to be dissipated. The issue in this area is not the helicopter at all, rather the pilot, or how quickly the human being can react. Much the same as the braking distance of an automobile is determined by two factors: how far the car travels while the driver is reacting by moving from the accelerator to the brake pedal, then how far the car travels once the brake is applied. For this portion of the H-V diagram the issue is how far will the helicopter drop before the pilot can react, because if it drops too far the aircraft can hit the ground or the pilot cannot flare. Consequently, it’s somewhat difficult to test since the test pilot who rolls the throttle off on himself will probably react quicker than the average pilot flying low along the beach on a warm summer day. Aft cyclic during the entry into the autorotation is especially important in this area of the chart to prevent an excessive rotor RPM decay.
OTHER CONSIDERATIONS
A night autorotation evaluation is also conducted. Autorotations to the ground are accomplished to evaluate any adverse glare from the outside aircraft lighting, cockpit lighting and the benefit of the landing light. If the aircraft is certified to fly over water, for example the R22 Mariner and R44 Clipper with fixed floats (not the R66 Marine because it is pop-out floats only) then a water evaluation is required. Selected points along the H-V curve are made to the water. If the aircraft is certified to fly over water at night, then a night water evaluation is conducted. The R22 Mariner is not certified to fly over water at night due to the navigation lights being blocked by the fixed floats, whereas, with the R44 Clipper the navigation lights were moved up on to the mast cowling so the Clipper completed a night water evaluation. Believe me, autorotations to the water – at night, are a little tricky. Interestingly, all H-V testing is completed using straight-in autorotations – no turns. So, in the real world if a turn is needed, a little more altitude and/or airspeed will be required. I believe one of the shortcomings of the rules is there is no requirement for the manufacturer to explain if a particular flight technique was used on various points. For example, on the low altitude/low airspeed points on the takeoff portion, was the collective slightly lowered or not. It was for Robinson’s three helicopters.
VARIABLES
One of the more important concepts to understand about the height-velocity diagram is that it is not cast in concrete. There are variables that make the shaded area get larger and ones that make the shaded area get smaller. With a good understanding of these variables pilots can be a little safer doing things they will probably do anyway but, as I say, perhaps a little safer. The first is density altitude. For a Part 27 approved diagram the manufacturer actually went up to 7000 feet density altitude and conducted an H-V test. We all understand that when the density altitude increases, aircraft performance decreases so we would expect the shaded area to get larger, but with a Part 27 diagram we actually see where and how much larger it gets. Weight is one of the more significant variables. The sea level test is done at maximum gross weight, but of course, we don’t always fly at max gross weight. Using the R44 Raven I chart above, how would the shaded area change if the flight was at 2000 lbs instead of 2400 lbs? There will be a decrease in the forward speed kinetic energy but since the formula is ½ M (or weight) the decrease will be small as long as the velocity (V²) is constant. However, the energy needed decreases on a linear basis as the weight decreases. In other words, it take twice as much energy to stop 2000 lbs from coming down as it does to stop 1000 lbs. So, at any point on the curve, if we compare the amount of energy available to the amount required that relationship gets better and better as the weight decreases making the shaded area get smaller and smaller. Something to remember when practicing autorotations, especially autorotations to the ground. Wind is another important variable. The test was done in a calm wind but what if there was a 20 kt headwind? Since the diagram is based on indicated airspeed (IAS), from an energy standpoint the glide portion of the autorotation would be the same. 50 kts indicated is 50 kts indicated – headwind, tailwind or no wind. However, once we begin to use the energy from that 50 kt glide in the flare that wind will continually replenish the system with ½ the weight X 50 kt² worth of energy all the way to the ground. So, as your experience would probably tell you, the shaded area will get smaller with a headwind and, conversely, larger with a tailwind. Needless to say, autorotations should be practiced into the wind. Individual pilot skill or proficiency is another variable. Depending on how often a pilot practices autorotations individual proficiency will vary. For example, a private pilot on the day of the private pilot practical test is probably very proficient with autorotations since that maneuver has been practiced continually leading up to the checkride (whether a high level of proficiency is demonstrated on the checkride is another question) as opposed to six months after the test with no autorotation practice. Autorotations are a real “use it” or “lose it” skill so recurrent training with an instructor is a must. As previously mentioned, turns and other maneuvering will also make the shaded area get larger.
Here are a few real-world examples of how a pilot can use these variables:
I am a brand-new commercial pilot and a photographer will actually pay me to take pictures from my Raven I. However, the photographer needs to hover out-of-ground effect at 350 ft. A quick glance at the H-V diagram tells me this is inside the shaded area and if the engine were to fail, I’ll have my hands full. Maybe I should have said “I’m a starving commercial pilot” – I need the money, so I will make this flight. What variables can I use to make this a little safer than it initially seems? Weight is probably the easiest to use – only take the fuel necessary, take the doors off or, if you’re really hard core, don’t eat breakfast. Perhaps there is some wind to take advantage of.
Now let’s say the photographer is not too concerned about altitude because of his telephoto lens but, because of the location of the sun needs to be headed in a direction I know will give me a tailwind. In this case I know the shaded area has increased so I will want to add sufficient altitude to accomplish a turn.
Density altitude, weight, wind, pilot skill and maneuvering all can make the shaded area expand or contract. Helicopters frequently need to operate inside the shaded area of the height-velocity diagram to complete their job which is why the diagram is located in the Performance Section of the Rotorcraft Flight Manual (RFM) not the Limitations Section (for Part 27 certification). Pilots should carefully consider each of these factors when operating in and around the shaded area.
One last comment and that’s about terminology. You’ll notice nowhere in this discussion is the term “Dead Man’s Curve” used. This well used but worn out term is not only technically incorrect but connotes something scary or menacing. The helicopter community needs to move past this kind of thinking and focus on reality. If you’re interested in a real “Dead Man’s Curve” listen to Jan & Dean’s 1964 hit song, which reached # 8 on Billboard’s Top 100 list.
Autorotation Bits & Pieces
Practice Autorotation or Simulated Engine Failure
First, I would like to make sure everyone is clear that there are two completely different maneuvers that involve autorotations. One, the “practice autorotation” and second, the “power failure at altitude” (sometimes called a forced landing, simulated engine failure or, my least favorite, a throttle chop). In training, each has a different purpose and all too often instructors try to combine them with confusing results.
The purpose of the “practice autorotation” is to teach the student the basics of autorotative flight. Fundamentals such as, attitude control, RPM control, turns, the importance of trim, where and how much to flare and, of course, the power recovery or touchdown are introduced and refined with practice autorotations. It’s not a surprise, in fact, the student normally enters the autorotation themselves making the maneuver quite different from the simulated engine failure. For details on practice autorotations see the discussion on the “basic autorotation” and “autorotation with turn”.
In the case of the “engine failure at altitude”, which is the term that will be used in the FAA’s new Airman Certification Standards (ACS) so; I guess I should use it now. In training, the purpose of the power failure at altitude is fourfold:
-
How to recognize an engine failure: Probably the most important of the four because if the pilot fails to recognize the engine failure everything else doesn’t much matter. Pilots might take this for granted but, you’d be surprised at the number of accidents, many fatal, where the pilot was not properly trained and reacted incorrectly with disastrous results. I talk about one in the Safety Course at Robinson where a newly rated pilot, whose instructor had never rolled the throttle off to simulate an engine failure, suffered an engine failure due to carburetor ice. When the engine failed and the helicopter kind of fell out from underneath the pilot, I suspect he responded by immediately increasing the collective because the rotor stalled at cruise altitude and the helicopter fell like a brick killing the pilot and his wife. Indications like the left yaw, loss of engine noise and low RPM warning have to be experienced and continually reinforced during training.
-
How to respond to an engine failure: Immediately entering an autorotation has to be an instinctive or intuitive response to an engine failure. There isn’t time to think it through, try to figure out what’s happening or pull out a checklist-you have to react. This required reaction can only be achieved by repetition. Instructors can talk on the ground until they are blue in the face about how important it is to lower the collective when the engine fails but it just doesn’t build the spontaneous type of reaction that’s needed. The engine failure has got to be simulated over and over, beginning in the pre-solo phase all the way to the private pilot checkride. (Obviously, in the pre-solo phase the simulation should be fairly benign. You don’t tell a pre-solo student to look over at the pretty sunset and as soon as the head moves snap the throttle off, but the foundation has got to be laid in this early phase.)
-
Select a landing area: (restated from the Enhanced Autorotation essay) When the engine quits and all the cockpit tasks are complete in transitioning to an autorotative glide, the first thing the pilot needs to do is select a landing area. Have a selection plan in mind, for example start at 9/10 o’clock and scan to 2/3 o’clock or do the reverse, perhaps look first into the wind then scan from there until a suitable site is found, but move your head. I see it over and over on a private and even commercial pilot check ride where I set the helicopter up with a large landing area 90º to the left or right, reduce the throttle to simulate the engine failure and the pilot never moves the head and picks some postage stamp size parking lot straight ahead.
-
Maneuver to the landing area: Use one or a selection of the enhanced autorotation techniques.
Certainly, numbers 1 & 2 are the most important because if these aren’t done properly the pilot never gets to 3 & 4.
The Power Recovery vs the Touchdown
The value of touchdown autorotation training has been the subject of constant debate within the helicopter community for as long as I can remember. One side argues the benefit the pilot receives in actually experiencing an autorotative landing and the opportunity to build proficiency in the technique greatly increases pilot confidence, thus reducing the chance of a catastrophic outcome to a real engine failure. They believe the power recovery aspect of the autorotation does not resemble the real situation and may even build a false sense of security on the part of the pilot. The other side claims the increased risk of damaging the aircraft with the touchdown maneuver is not worth the benefit gained over terminating with power at a hover. They feel that with the increased reliability of today’s modern engines, the helicopter community will damage/crash more aircraft practicing for an event that rarely occurs.
The FAA Practical Test Standards (PTS) do not require the applicant to demonstrate proficiency at touchdown autorotations during the private, commercial or ATP practical test. Instead, preferring a power recovery as appropriate. Until 2016 the Flight Instructor Practical Test Standards did require a CFI applicant to demonstrate proficiency in touchdown autorotations. The thinking being the flight instructor, who is frequently splitting the needles with practice autorotations, is much more at risk to actually have to perform a real autorotation and I certainly agree with this thinking. In March 2016 the FAA modified their stance on the touchdown for the CFI to be very similar to the way the airplane community treats spins; The examiner may accept a logbook endorsement stating the CFI applicant has received training in touchdown autorotations and has demonstrated competence in lieu of actually doing one on the checkride. The key word here is may, it’s up to the examiner. I tell my initial CFI applicants to plan to do a touchdown on the test but bring an endorsement in case conditions on the day of the test (wind direction, speed, aircraft weight etc.) are not conducive to touchdowns. This way I hope to avoid the situation that existed back in the 1980s when I became an examiner. At that time, only a logbook endorsement was required and the examiner had no option but to accept it. Consequently, the way things would typically happen is a few days before the checkride the applicant would go up with their instructor, throw a few autos onto the ground, get the endorsement and head for the test. Having seen a few touchdowns is nowhere near being competent. As far as the private, commercial and flight instructor tests are concerned I think the present day balance between the three is good. The future Airman Certification Standards (ACS) plans to maintain this balance with one minor change. The new ACS will allow a touchdown autorotation on the private or commercial checkride if both applicant and examiner agree whereas under the PTS a power recovery is required.
FAR Part 135 initial and recurrent training, also, does not require a touchdown autorotation. The FAA does conduct touchdown autorotation training for FAA inspectors during their helicopter recurrent training using the same logic as with the flight instructors. At the Robinson Safety Course we do a lot of touchdown autorotations but only under a strict set of circumstances; wind direction, wind speed, half fuel and a hard surface. Most law enforcement agencies and many commercial operators require touchdown autorotations during recurrent training but it’s a very common practice to contract out this training rather than perform the maneuver in their own aircraft. Throughout the 1960s, 70s and most of the 80s the US Army conducted only touchdown autorotation training for both initial aviator qualification and yearly recurrent training. However, in the late 1980s the Army eliminated touchdown autorotations for all but initial training due to an excessively high accident rate. Now with the Army’s initial entry trainer being the twin engine UH-72 Lakota, all autorotation training, power recovery and touchdown, will, in my view, suffer.
Teaching touchdown autorotations requires a high degree of experience and proficiency on the part of the instructor. I recommended these autorotations be practiced only with an instructor experienced in the particular make and model and current in performing touchdown autorotations.
Grass vs Hard Surface
When it comes to the landing surface for touchdown autorotations, there’s nothing like a fresh mowed, well drained, level grass runway. It’s almost a cushioning effect on touchdown without the jarring, scraping noises you get on concrete. Europe, especially the UK, has many more of these well maintained, iconic grass striped aerodromes, many left over from World War II, than exist here in the US. It’s like being in a time machine to look down on these airfields from the traffic pattern and visualize a squadron of spitfires beginning their takeoff roll down an emerald green runway. However, when it comes to touchdown autorotations, make no mistake about it, there is no question a hard-surfaced runway or taxiway is much, much safer. The grass surface works fine if you do everything perfectly but, the problem is, not every autorotation is perfect, particularly when training pilots new to touchdown autos. If a grass runway or soft surface is the target, closely inspect it before using it each day because, unlike a paved surface, its conditions can change overnight. Test the surface by touching down from a hover moving forward, power-on to evaluate the condition of the landing surface.
I’ve seen several reasons cited for the preference of a paved surface over a grass or soft surface but I think two stand out above the rest. First and foremost, is the paved surface allows the skid gear to slide along the surface if the landing is made with any sideward movement or the skids are not parallel to the ground track. The soft surface can inhibit this sliding setting up a classic dynamic rollover scenario. In my experience, landing with the skid gear not parallel to the ground track is the most common pilot error on touchdown. Failing to correct for a crosswind, failing to correct for the yaw caused by friction through the drivetrain when the collective is increased or just plain poor pedal control can all lead to a sideward movement on touchdown. I was turned almost 90˚ once when my student in a UH-1 applied left pedal (instead of right) at the bottom of an autorotation but we just slid sideways to a stop because of the paved surface–a sure rollover had we been on grass. Second, the soft surface can cause a more sudden deceleration after touchdown and in combination with the generally high center of gravity pitches the nose down. The natural pilot reaction is aft cyclic but if the rotor RPM is too low, a blade can flap down striking the tail cone.
So, put up with the noise, the jarring feeling and let the “spring and yield” design of the skid gear work as it’s supposed too. At Robinson we require all touchdown autos be done to a paved hard surface- runways, taxiways but, hopefully, not freeways.
What About a Mayday Call?
I’ll bet for every 100 private, commercial and even flight instructor practical tests I give, during the simulated engine failure I’ll be lucky to hear 3 simulated mayday calls. It’s just not taught by most of the US helicopter schools and instructors. Contrast that with my US Army training where on a forced landing (Army term) the mayday call was required, meaning if you skipped the mayday call you failed the maneuver. Give a surprise engine failure to an Army pilot, which I’ve done thousands of time in my 20+ years as an Army instructor pilot, and they’ll have three maydays out of the mouth before the collective is full down. So, I’m on a one-man crusade to make the “mayday call” or some might call it a “distress call” an integral part of US civilian autorotation training (Civilian training outside the US does a much better job.). To this end, I’m pushing hard as part of the FAA Airmen Certification Standards Working Group to make the mayday call a required skill for the task “power failure at altitude” and to include a discussion in the revised Helicopter Flying Handbook. We’ll see how successful I am?
What does a mayday call consist of and when should it be made? The mayday call comprises four components:
-
Transmit “mayday, mayday, mayday” over the radio (in some parts of the world it’s “pan, pan, pan”)
-
Who you are.
-
Where you are.
-
Nature of the emergency i.e. engine failure, fire, etc.
Number 2 is not too difficult as most pilots know their N number and number 4 should be easy, but it’s number 3 that can be tough. Remember, you’re trying to give a position that will really help people find you so it has to be accurate and specific. For example, “35 miles south of Los Angeles” probably won’t help much, there’s a lot of territory 35 miles south of LA. It’s a critical situation where the pilot has to fly, think, and talk all in the midst of a crisis. If you haven’t practiced the mayday call, you’ll chew your tongue up trying to get a good call out in the heat of battle coping with a real emergency.
When should the call be made? For the most part it’s up to the pilot to determine when a mayday call is needed. Certainly any power off or “land immediately” procedure will most likely necessitate making one. Once the decision to make a mayday call is made where in the emergency sequence should it be attempted? Remember the old “first fly the aircraft” mantra. So first take care of any immediate concerns for example, when the engine fails take care of all the important cockpit duties i.e. enter the autorotation, select a landing area, determine your maneuvering plan then make the mayday call. If you have power but in a “land immediately” situation time is not quite as critical so determine how the emergency will be handled then make the mayday call.
No-flare Autorotations
The “no-flare” or “constant attitude” autorotation has been around for years however, I am not a big fan of it. The US Army taught no-flare autos during the 1960s in the OH-13 model and the 1973 FAA Basic Helicopter Handbook (AC 61-13A) describes it as one of the standard autorotation techniques. My first job as a CFI (1973) was in a Bell 47-D1 and we used the no-flare auto as a preparatory maneuver before introducing the flare auto. In the UK the technique is taught as the best option for night autorotations because of the difficulty judging the proper flare altitude and some suggest it should be used in IMC (instrument meteorological conditions).
After the entry into an autorotation, the technique is to reduce to a 30-40 kt attitude which will be a somewhat level attitude in most helicopters. Maintain this attitude during the glide, at 50 ft insure a landing attitude and at approximately 10 ft increase the collective as necessary to cushion the landing. Sounds pretty straightforward and simple, well, the problem is little room for error exists. From an energy standpoint, at this slow airspeed there is little kinetic energy to arrest the descent rate so all the energy needed to prevent spreading the skids or worse is in the rotor RPM, which makes timing the collective increase critical. Somewhere around 100 ft AGL the sensation of the ground rushing up becomes a bit startling and increases the lower the aircraft gets. When I did this in the high inertia Bell 47 at moderate weights and maybe a little wind, it wasn’t too difficult to judge. However, with a lower inertia rotor at the higher weight ranges (i.e. a Robinson R22 with two on board) the margins are very slim. Even the R44 or Bell 206 with a load of passengers will be tricky, especially in a calm wind. In my view if you have the altitude to trade for airspeed then use the energy from that speed in the flare you’re much better off.
So are no-flare autos appropriate at any time? For the IMC situation I mentioned above, I’m assuming the proponents mean on an actual instrument flight not an inadvertent IMC situation, because if the engine fails shortly after inadvertently punching into the weather the best move is probably to put your hands together underneath your chin and pray–because it’s not your day. On an actual instrument flight I believe after entering the autorotation it’s best to transition to “minimum rate of descent configuration” (Vy and the lowest allowable rotor RPM for autorotation). Rarely do the clouds extend from the MEAs (minimum enroute altitude) all the way down to the surface so you’ll exit the clouds descending at the slowest rate and, hopefully, have time to select a landing area. We frequently practiced this under the hood in the Army. For the night situation I still feel the flare autorotation is best. Both will be tricky if the landing area is not lit but, at least the flare auto is something most pilots have practiced (in many cases practiced at night with a power recovery) and in the heat of battle when the pressure is on relaying on training is preferable to attempting a technique rarely practiced. There is one instance I can think of where the no-flare technique might be best and that’s over heavily wooded terrain with only small clearings available so a more vertical descent angle is needed. Here the combination of the small area and tall trees make the flare autorotation much more problematical and most likely result in excessive descent rates because of the height of the flare (above the trees). However, if the area is large enough to fit two or three aircraft, it’s probably large enough for the flare auto using the enhanced autorotation techniques.
Night Autorotations
I’m sure every pilot would agree autorotations at night can be a real challenge and to be honest a little scary. Real important things like judging altitudes, descent rates and determining the wind are so much harder because you just can’t see as well so many of the visual cues you use during the day don’t exist at night. I remember in the Army during the 70s and 80s all pilots had to perform night autos semiannually. We did straight-ins, 180˚s and, believe it or not, low level autos (entry from 100 feet at 90kts) all to the ground at night. Since an instructor pilot (IP) had to be at a set of controls for all autorotations, day or night, I would find myself out there four or five times a month riding through these night autos while the unit’s pilots were getting their minimums. I never got comfortable seeing the sparks fly as the tungsten carbide skid shoes scraped along the runway. Thankfully, night vision goggles (NVG) made their way into the Army inventory and by the late 80s unaided night autos were a thing of the past.
On the civilian side night touchdown autorotations are almost never practiced but power recovery autos at night are not uncommon even at the private pilot level. As a part of the FAA aircraft certification requirements of 14 CFR§ 27, we conduct a night touchdown evaluation to determine any adverse glare from cockpit lighting and outside lighting including the landing light. However, these tests and for that matter my Army night autos were always done to a lighted runway. In fact I would frequently call the tower to make sure the intensity of the runway lighting was on high so the peripheral lighting provided by the runway lights substituted for the landing light that points towards the stars during the flare. Therefore some training organizations prefer the no-flare auto at night and one country, I believe the UK, at one time required flares that would be fired to illuminate the landing area for commercial helicopters without a moveable landing/searchlight. Obviously, in the real world you won’t be able to just key the mike 5-7 times to light the landing area up.
Bottom line is autorotations at night are tricky. If you must fly at night, make the following adjustments; plan your route to take advantage of ground lighting forsaking direct routes, use much higher en route altitudes and, probably the most important, maintain a high degree of night currency. As a last resort remember the old Army adage; “if the engine fails at night turn the landing light on. If you don’t like what you see, turn it off”.
Autorotations to Water
Over the years I have taught 10 or 12 Robinson Safety Courses for two major tuna boat companies in Mexico who fly the R44 Clipper on fixed floats. I’ve always enjoyed the opportunity to get back in a Clipper and reacquaint myself with the flight characteristics of the floats both in flight and on the water. I will divide this discussion up into autorotations to water with fixed or pop-out floats and, what would probably best be described as “ditching power-off”, that is autorotations to water without floats.
14 CFR § 27 requires a power-off water evaluation if the helicopter is approved for over water flight and if the approval includes over water flight at night, a power-off night water evaluation is required. For example, the R22 is not certified for over-water flight at night because of the location of the position lights (blocked by the fixed floats), whereas, the R44 Clipper is approved for over-water flight at night (position lights are moved onto the mast cowling) and completed a power-off night water evaluation. From an autorotation standpoint, there is not much difference between fixed floats and pop-out floats other than the obvious question of “when to pop the floats”. Assuming a cruise condition, I feel it’s best to get the floats deployed as soon as possible after establishing the autorotation, slowing below the power-off Vne and, if necessary, turning into the landing direction. This way the pilot can assess a satisfactory deployment and, at least mentally adjust or prepare for any problems with a partial deployment. Getting any turn out of the way prior to deploying the floats is preferable because of the adverse roll characteristic of helicopters with floats (The tendency to roll in the opposite direction of the turn if sideslipping and requires a little pedal in the turn's direction to maintain the aircraft in trim.) that the fixed float pilot is probably familiar with but will be new to the pop-out float pilot. The biggest challenge in an autorotation to water is judging the height above the water for the flare and collective increase at the bottom. Smooth water can be especially difficult but even choppy water has issues so try to use a shore line, boats or other visible objects to determine height. Touchdown should be in a slight nose high attitude or heels first to prevent the toes of the floats from submerging causing the helicopter to nose over. After touchdown hold the cyclic in the touchdown position and do not lower the collective until all forward movement has stopped.
An autorotation to water without floats is certainly a product of poor planning, bad judgement and not much luck, but it happens. If possible, jettison the doors/windows or at least unlatch the doors. The idea here is to allow water in after contact so the pressure from the water equalizes making an exit through the doors possible. Studies have shown that small and mid-sized helicopters, without large spaces to trap air enabling the aircraft to float, commonly invert and quickly sink making a speedy egress extremely important. Consequently, a source of ongoing discussions, bordering on arguments in many cases, among pilots is which way to roll the helicopter upon contact with the water. The variables are so diverse there is not a text book solution; however, a little common sense might help. Let’s agree you should not exit the helicopter until the blades stop spinning so the goal is to get the blades into the water as soon as possible and use the water to slow the rotation. This being the case, I think the pilot should roll the aircraft in the direction it wants to go so the blades enter the water shortly after the skids. Clearly, if the helicopter rolls to the right but the pilot has full left cyclic applied, blade contact with the water is delayed. Crosswinds, lateral movement, wave size/direction, tail rotor thrust or which skid enters the water first (lateral center of gravity) can all make the helicopter go one way or the other. If it wants to go left, roll it left and if it wants to go right, roll it right. As I said previously, there is not a text book answer but having twice been through the US Navy’s “dunker” training (underwater escape training at NAS Miramar, CA) I can say this with assurance “exiting a sinking helicopter after a crash will be a frightening experience”. If you fly over water without floats frequently, go back and read the first sentence of this paragraph.
One last point on autorotations to water; when the engine quits, you will have less than a minute to brief any passengers on what they should or shouldn’t do. So, any over-water flight requires a detailed preflight passenger briefing. Read the NTSB accident report, ERA12MA005 to understand how important an over-water passenger briefing is.
Autorotation is the Last Resort
As a result of a number of factors, it seems helicopter pilots have developed an almost supernatural dependence on the autorotation. In the back of their minds, the autorotation is the tool that will get them out of whatever predicament the aviation gods have thrown at them. Nothing could be further from the truth. Instead of the first, it should be the very last resort the pilot should turn to. Every effort should be made to maintain RPM and only when it’s no longer possible should an autorotation enter the picture.
Through the years I’ve seen accident after accident where the pilot prematurely entered an autorotation that ended with a less than satisfactory outcome. A well-known Army UH-1 accident comes to mind. The pilot sitting in the right seat reached with his left hand to adjust something on the center console, as he returned his arm the Velcro wrist cuff on his flight suit caught the governor beep switch, decreasing the RPM until the low RPM warning system activated. Thinking the engine had failed; the pilot immediately entered an autorotation, turned towards a landing area and eventually spread the skids at the bottom. A quick scan of a few instruments would have told the pilot the engine did not quit and, in this case, regaining the RPM is a simple procedure. Or the R22 pilot who had one of the two drive belts fail. The clutch light came on and stayed on indicating the problem. The pilot followed most of the emergency procedure correctly; he reached down, pulled the clutch circuit breaker but instead of a powered approach and landing (the R22 will fly on one belt) the pilot entered autorotation and chopped the tailcone off on touchdown. The RPM had never left the green range.
I think this seed of an autorotation being the “cure all” maneuver is planted early in a pilot’s training when autorotations are introduced and is continually reinforced by the constant focus on autos during the training. The day before or the flight before a private pilot test, what do you think is the one maneuver being practiced over and over? Correct, autorotations. Whenever I transition pilots into a new helicopter model, it’s not the normal approach that gets their attention, it’s autorotations. Sure, the autorotation is probably the quickest way to the ground and there are certainly times when we want to get to the ground quickly, but autorotative flight introduces a whole host of elements that may be difficult to digest in one’s eagerness to get to the ground. Do everything you can to maintain that RPM; reduce power, slow down, work the throttle, apply carb heat and only when all else fails… enter autorotation.